How to chill an object by sending its heat into space
Scientists demonstrate a cool new technique for getting rid of unwanted warmth
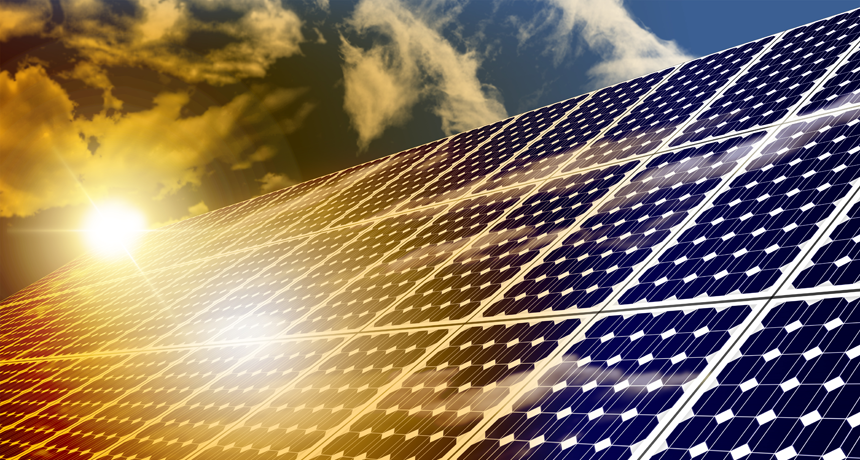
Solar panels collect energy from space. Now researchers have built a device that works in reverse: It cools an object by radiating its energy into outer space.
EzumeImages /iStockphoto
By Sid Perkins
When a refrigerator cools your food, it takes the heat away and dumps it into your kitchen. That adds to your home’s cooling bills. Likewise, when your air conditioner cools your home, it sends that heat outdoors. It also makes things warmer for everyone else in your neighborhood. The farther away you can send heat, the better. And there’s not much farther you can send it than outer space. Now, researchers have built a device to do just that. It cools down an object by radiating its heat directly into space.
For now, the device isn’t too practical. But its designers say that such cooling methods, combined with other techniques, might one day help people get rid of unwanted heat. The device would be especially well suited for arid regions, they add.
Radiation is the means by which electromagnetic waves carry energy from one place to another. This energy might be starlight traveling through space. Or it could be the heat of a campfire warming your hands.
The bigger the temperature difference between two objects, the quicker that heat energy can radiate between them. And not many things are colder than outer space, notes Zhen Chen. He’s a mechanical engineer at Stanford University in Palo Alto, Calif.
Outside of the envelope of gases surrounding Earth — our atmosphere — the average temperature of space is about –270° Celsius (–454° Fahrenheit). Chen and his team wondered if they could take advantage of this big temperature difference between Earth’s surface and outer space to cool an object on Earth, using radiation.
For an object on Earth to shed energy to space, radiation must travel through the atmosphere. The atmosphere doesn’t let all wavelengths of radiation through, Chen points out. But certain energy wavelengths can escape with little resistance.
One of the atmosphere’s clearest “windows” is for wavelengths between 8 and 13 micrometers. (At these wavelengths, electromagnetic radiation is invisible to the human eye. Because their energy is lower than that of red light, these wavelengths are called infrared.) Fortunately, says Chen, objects at about 27 °C (80.6 °F) radiate much of their energy in just that window.
Building a heat-emitting device
To study the new concept, Chen’s team built an object they would try to cool. They used mostly silicon. The basic ingredient in beach sand, silicon is both cheap and sturdy. It’s also the material computer chips are made from. That meant Chen’s team could use the same techniques used in making computer chips.
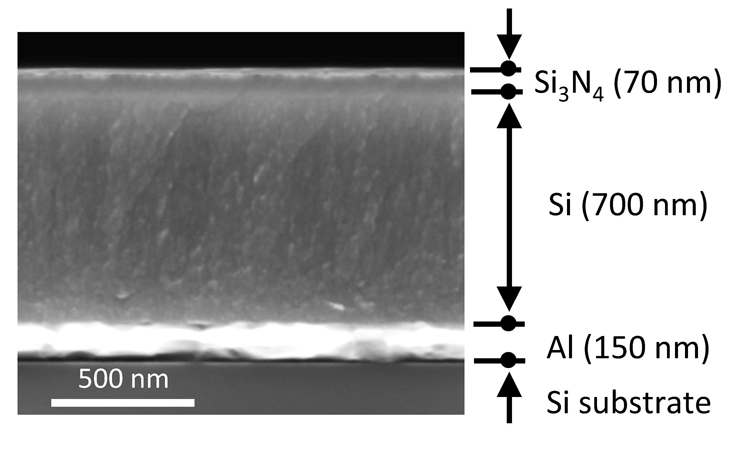
The base of their object was a super-thin disk of silicon, about twice the thickness of a human hair. That layer was for structural support. To that, they added a thin layer of aluminum. It reflected light waves like the shiny layer on the back of a glass mirror. The aluminum layer would send the object’s heat upward, toward space.
Next, the researchers added the layer of material they wanted to cool. It, too, was made of silicon, but was much thinner than the base layer. It was just 700 nanometers — billionths of a meter — thick. Finally, they coated the object’s upper surface with a 70-nanometer-thick layer of silicon nitride. The researchers chose that material because it mostly emits radiation in the 8- to 13-micrometer wavelength range. That means much of the heat energy from an object coated with this material could pass through the atmosphere and into space.
To accurately test their heat-radiating device, the researchers had to make sure the silicon disk couldn’t give off or soak up energy any other way.
Radiation isn’t the only way objects can transfer energy. Another way is conduction. It happens as atoms move around and bump into one another. During this natural jostling, warmer atoms transfer some of their energy — heat — to colder atoms.
To minimize energy transfer through conduction, Chen and his team built a special chamber to hold their disk. Inside, they placed the disk on top of four small ceramic pegs. The result was kind of like a tiny table. Ceramics do not transmit heat well. So with this design, very little heat could move from the disk to the chamber floor through conduction.
The researchers also wanted to minimize heat loss through convection. That’s where an object transfers heat to the air or liquid around it, allowing that fluid to heat nearby objects. To make sure that their disk’s heat wouldn’t be lost by convection, Chen’s team sucked all the air out of the chamber.
The only remaining way for the object to lose heat was through radiation.
Next, the researchers took steps to make sure the disk didn’t gain heat from its surroundings. That meant minimizing the radiation that could reach it from the outside. First, they made the upper surface of the chamber (the one pointed toward space) from a special material: zinc selenide. This material only lets in radiation between the wavelengths of 8 and 13 micrometers.
The team also designed a special panel that blocked sunlight and kept the chamber in the shade during tests. This kept the object from absorbing heat directly from the sun. They also put a cone of reflective material around the top of the chamber. That would help stop gas molecules on the object’s sides from radiating their heat to it. They left a window straight up to space for the object’s heat to escape.
An “extreme experiment”
The team tested its device on the roof of their building at Stanford. Some of those tests spanned a full 24 hours. The object’s heat energy successfully disappeared into space. This radiant loss of heat could cool their object by an average of 37 degrees C (67 degrees F).
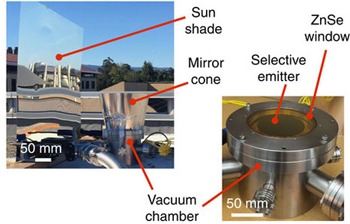
As Chen expected, moist air in the atmosphere reduced the system’s effectiveness. His team had known that water vapor blocks some radiation in the normally clear 8-to-13-micrometer window. But the cooling indeed was efficient when the humidity was low.
Chen’s group described its work December 13 in Nature Communications.
The team’s cooling tests “are an extreme experiment that demonstrates the possibility” of cooling objects by radiating their energy to space, says Geoff Smith. He’s a physicist at the University of Technology Sydney in Australia.
But the cooling device the team built isn’t exactly a useful refrigerator, he adds. For one thing, the object the team cooled is small and specially designed. If the team instead tried to cool something like a can of soda, “it would take them a long, long time,” he says.
“It’s hard to see how this could be a primary method of dumping energy,” Austin Minnich agrees. He’s a materials scientist at the California Institute of Technology in Pasadena. In other words, a cooling device like the team’s prototype might not be able to cool something all by itself. But it could help out other types of cooling systems, Minnich suggests.
That extra help might be a little bulky, though. For one thing, he notes, to radiate energy at the same rate as a 100-watt light bulb, engineers would need to build a surface of about 1 square meter (10.8 square feet). That’s about the same size as some rooftop solar panels.
Chen acknowledges that the team’s cooling device is small. And sometimes engineers have problems making experimental devices work when they try to enlarge them. One challenge to making the heat-shedding device bigger is that the chamber it’s in needs to be airless (a vacuum). Sucking all the air out of a larger chamber without making its walls crumple is tricky.
Another hurdle to enlarging the team’s device is cost, notes Chen. In particular, zinc selenide (the material the team used as the top of their cooling device) is quite expensive. But with further research, he says, engineers might find a cheaper substitute.