It took a ‘virtual’ telescope to actually picture a black hole
The accomplishment relied on a network of radio telescopes spanning the globe
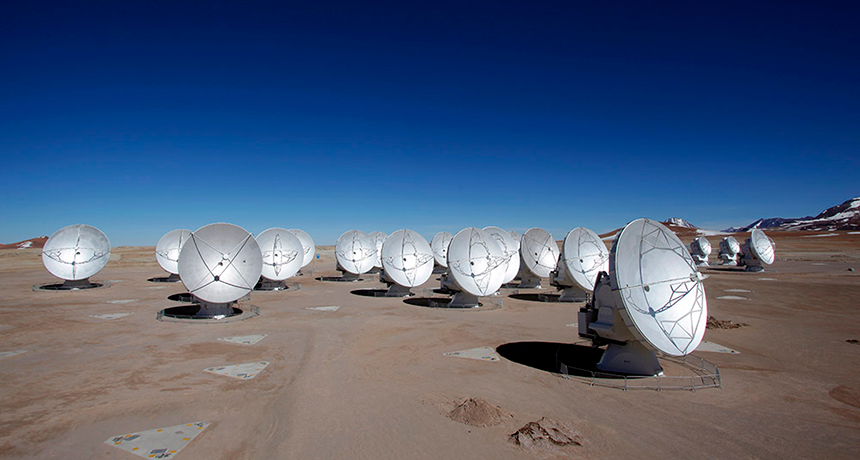
A network of telescopes across the globe — including ALMA in Chile (partially shown here) — teamed up to create the Event Horizon Telescope. It’s a virtual radio dish almost as big as Earth.
Carlos Padilla/AUI/NRAO
Black holes are camera shy. Their extreme gravity prevents light from escaping. That means that the dark hearts of these cosmic heavy hitters remain entirely invisible. However, supermassive black holes in the centers of galaxies may give themselves away by spewing bright jets of charged particles. Others may be “seen” by the light of the nearby stars that they fling away or rip apart. Up close, these behemoths are surrounded by accretion disks — glowing disks made from the material being sucked into them.
Scientists have now cleverly created a network of eight radio telescopes. Working as one, they effectively make an Earth-sized eye on the sky. And they have just imaged the silhouette of a black hole’s event horizon — the edge inside which nothing can be seen or escape. It can be seen against the black hole’s accretion disk.
In April 2017, this so-called Event Horizon Telescope, or EHT, collected data that have now yielded the first image of a supermassive black hole. It sits inside the galaxy M87.
“There is nothing better than having an image,” says Avi Loeb. He’s an astrophysicist at Harvard University in Cambridge, Mass. Though scientists have collected plenty of indirect evidence for black holes over the last half century, he notes that “seeing is believing.”
Creating that first-ever portrait of a black hole was tricky, though. Black holes take up a minuscule sliver of sky. And they’re so far away that the halo of light surrounding some of them appears very faint. The project of imaging M87’s black hole required eight observatories across the globe. By working as one virtual radio dish, their vision would be sharper than that of any single observatory working on its own.
Putting the ‘solution’ in resolution
The supermassive black hole inside M87 is no small fry. It weighs in at around 6.5 billion times the mass of our sun. But viewed from 55 million light-years away, it appears as the smallest of blips in the sky. It’s smaller than an orange on the moon as viewed by someone on Earth. Still, besides Sagittarius A* — the black hole at the center of our own galaxy — M87’s black hole is the largest black hole silhouetted on the sky.
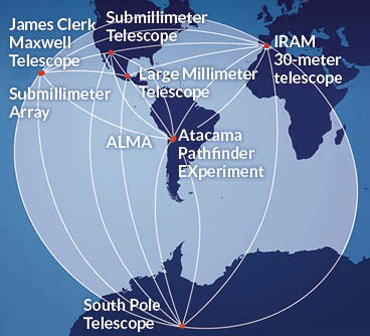
Only a telescope with EHT’s resolution could pick out something so tiny. A telescope’s resolution depends on its diameter: The bigger the dish, the clearer the view. So getting a crisp image of even a supermassive black hole needed a planet-sized radio dish.
“The trick is that you don’t cover the entire Earth with an observatory,” explains Loeb, who wasn’t involved in EHT. Instead, astronomers combine radio waves seen by many telescopes at once. This makes the telescopes effectively work as one giant dish. The diameter of that virtual dish is equal to the length of the longest distance, or baseline, between two telescopes in the network. For the EHT in 2017, that was the distance from the South Pole to Spain.
Telescopes, assemble!
The EHT was not always the hotshot array that it is today. In 2009, a smaller network of just four observatories — in Arizona, California and Hawaii — imaged the base of one plasma jet spewing from the center of M87’s black hole. But this small telescope network didn’t yet have the magnifying power to reveal the black hole itself.
Over time, the EHT program recruited new radio observatories. By 2017, there were eight observing stations in North America, Hawaii, Europe, South America and at the South Pole. Among the newcomers was the Atacama Large Millimeter/submillimeter Array, or ALMA. It is located on a high plateau in northern Chile. With a combined dish area larger than an American football field, ALMA collects far more radio waves than other observatories.
“ALMA changed everything,” says Vincent Fish. He’s an astronomer at MIT’s Haystack Observatory in Westford, Mass. It can offer “really solid detections now,” he says, of “anything that you were just barely struggling to detect before.”
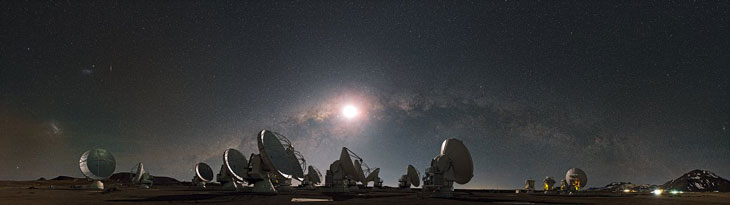
More than the sum of their parts
EHT observing campaigns are best run within about 10 days in late March or early April. That’s when the weather at every observatory promises to offer the clearest images of the sky. Researchers’ biggest enemy is water in the atmosphere, such as rain or snow. It can muddle the millimeter-wavelength radio waves to which the EHT’s telescopes are tuned.
Planning for weather on several continents can be a headache.
Geoffrey Bower works at the Academia Sinica Institute of Astronomy and Astrophysics in Hilo, Hawaii. “Every morning” during an EHT campaign, this astronomer notes, “there’s a frenetic set of phone calls and analyses of weather data and telescope readiness.” Afterward, “we make a go/no-go decision for the night’s observing.” Researchers are picky about conditions. But toward the tail end of the run, they’ll take what they can get.
When the skies are clear enough to observe, researchers steer the network’s telescopes at each EHT observatory toward the vicinity of a supermassive black hole. Then they begin collecting radio waves. M87’s black hole and Sgr A* appear on the sky one at a time. Each one is just about to rise as the other sets. This allows the EHT to switch back and forth between observing its two targets over the course of a single multi-day campaign. All eight observatories can track Sgr A*. Because M87 is in the northern sky, it can’t be seen by the South Pole station’s sight.
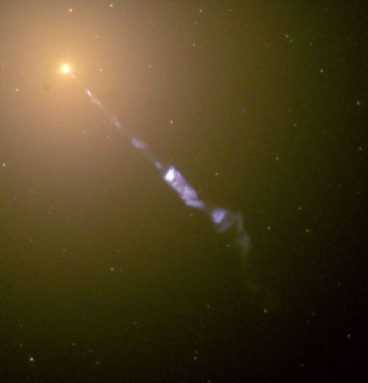
On their own, the data from each observing station look like nonsense. But taken together, these data can reveal a black hole’s appearance.
Here’s how it works. Picture a pair of radio dishes aimed at a single target. In this case, it’s the ring-shaped silhouette of a black hole.
Radio waves emanating from each bit of that ring must travel slightly different paths to reach each telescope. These radio waves can interfere with each other. Some will reinforce one another. Others will sometimes cancel each other out. The pattern of waves seen by each telescope depends on how the radio waves from different parts of the ring are interacting when they reach that telescope.
For simple targets, such as individual stars, the radio waves picked up by just two telescopes can provide enough data for researchers to figure out how the light is distributed across the sky. But a black hole is a complex light source. There are too many possible solutions for what the image could be. So researchers need more data to work out how a black hole’s radio waves are interacting.
The ideal array has as many baselines of different lengths and orientations as possible. Telescope pairs that are farther apart can turn up finer details. That’s because there’s a bigger difference between the pathways that radio waves take from the black hole to each telescope. EHT includes telescope pairs with both north-south and east-west orientations. And as Earth rotates, these change, relative to the black hole.
Pulling it all together
In order to braid together the observations from each observatory, researchers need to very precisely record the time they collect their data. So the astronomers use atomic clocks (ones that lose about one second every 100 million years).
There are a lot of data to time stamp. “In our last experiment, we recorded data at a rate of 64 gigabits per second.” Explains Bower, that’s “about 1,000 times [faster than] your home internet connection.”
These data are then transferred to the MIT Haystack Observatory and the Max Planck Institute for Radio Astronomy in Bonn, Germany. There, the data are processed in a special kind of supercomputer. It’s called a correlator.
Each telescope station amasses hundreds of terabytes of information during a single observing campaign. That’s far too much to send over the internet. So the researchers use the next best option: snail mail. So far, there have been no major shipping mishaps. Still, Bower admits that mailing the disks is always a little nerve-wracking.
Although most of the EHT data reached Haystack and Max Planck within weeks of the 2017 observing campaign, there were no flights from South Pole until November.
Filling in the blanks
Combining the EHT data still isn’t enough to render a crisp image of a supermassive black hole. If M87’s black hole were a song, then imaging it the EHT data would be like listening to the piece played on a piano with a bunch of broken keys. The more working keys — or telescope baseline pairs — the easier it is to get the gist of the melody.
“Even if you have some broken keys, if you’re playing all the rest of them correctly, you can figure out the tune,” says Fish. “That’s partly because we know what music sounds like,” he notes. “The reason we can reconstruct images, even though we don’t have 100 percent of the information, is because we know what images look like” in general.
There are math rules about how much randomness any given picture can contain — such as how bright it should be and how likely it is that neighboring pixels will look similar. Those basic guidelines can inform how computer software decides which data interpretations make the most sense.
Black holes and beyond
EHT’s black hole observations are expected to help answer questions like how some supermassive black holes, including M87’s, launch such bright plasma jets. Understanding how gas falls into and feeds black holes could also help solve the mystery of how some black holes grew so quickly in the early universe, Loeb says.
Loeb says the EHT also could be used to find pairs of supermassive black holes orbiting one another. Two such relatively small black holes collided, creating the gravitational waves detected in 2015. Getting a census of such black-hole pairs may help researchers identify targets for the Laser Interferometer Space Antenna, or LISA. It’s goal will be to search from space for gravitational waves kicked up by the movement of objects like black holes.
EHT has few viable targets other than supermassive black holes, says Daniel Marrone. He’s an astrophysicist at the University of Arizona in Tucson. There are few other things in the universe that appear as tiny but bright as the space surrounding a supermassive black hole. “You have to be able to get enough light out of the really tiny patches of sky,” explains Marrone. “In principle, we could be reading alien license plates or something,” but they’d need to be super bright.
Too bad for alien seekers. Still, spying supermassive black holes is a pretty neat trick.
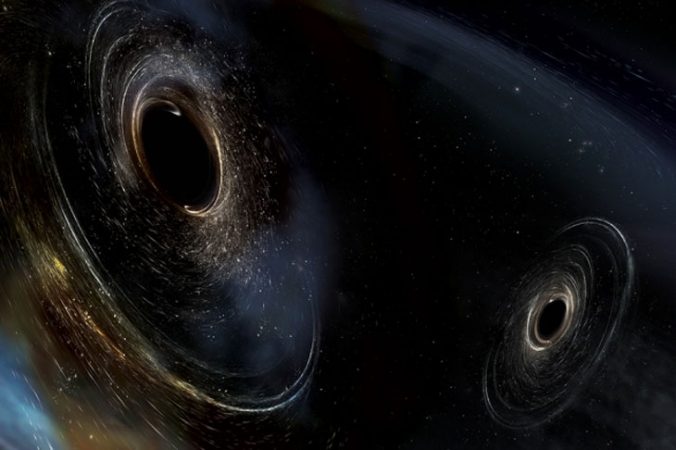