What scientists hope to learn from Great American Eclipse
The August 21 event offers a perfect chance to investigate big questions about our star
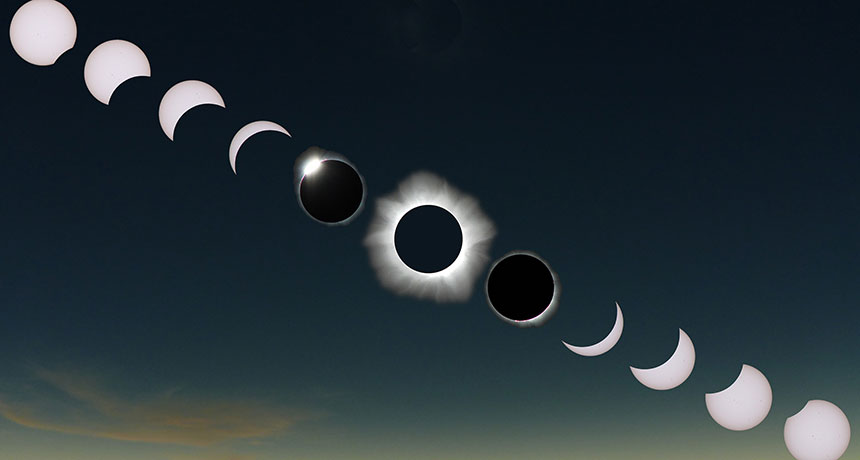
This time-lapse photo shows the stages of a November 2012 solar eclipse in the South Pacific.
RICK FIENBERG/TRAVELQUEST INTERNATIONAL/WILDERNESS TRAVEL (CC BY-NC-ND 4.0)
A total eclipse of the sun is not all that rare. One is visible from somewhere on Earth every 18 months or so. Most, however, go almost entirely unseen. Often the narrow zone of totality — where the sun is completely blocked out — falls over the oceans. Or cloud cover blocks precious minutes of totality over land.
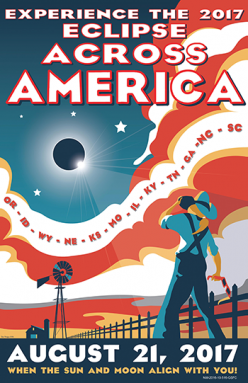
But this year’s Great American Eclipse, on August 21, will offer 93 minutes of totality on solid ground. (Each location gets about two minutes). That means more chances for land-based observers to have clear skies. It also provides a rare opportunity. For the full 90 minutes it takes the eclipse to travel from Oregon to South Carolina, people can watch the dance and sway of the sun’s corona. That’s the outer edge of the atmosphere jacketing the star.
With the sun’s otherwise blazing light covered up, that atmosphere suddenly becomes visible. Loops and whorls of plasma — energetic streams of charged subatomic particles — can be seen flying out from the sun’s surface. Many double back or twist and bend.
Not surprisingly, this corona will be a featured spectacle of this year’s eclipse.
In fact, that momentary solar shadowing will be much more than a spectacle. As the moon passes in front of the sun, scientists will be doing some serious work. A fleet of instruments will turn skyward to study parts of our star that usually are invisible.
But is there really any science left to do? Historically, eclipses were the only way to get a good look at parts of the sun. These days, space telescopes monitor the sun in multiple wavelengths, 24/7. So what else is there to learn?
A lot, it turns out.
“Everybody’s taken in by the beautiful dark sky” of an eclipse, says Padma Yanamandra-Fisher. “But that part is so dominant that people don’t always appreciate the nuances of the science you can actually do.” Yanamandra-Fisher is an astronomer at the Space Science Institute’s branch in Rancho Cucamonga, Calif. She will observe the event from Carbondale, Ill.
Many of the answers that she and other researchers seek have to do with the inner corona. It’s the part of the sun’s atmosphere that starts right at the solar surface and extends on out to about 2.5 times the radius of the sun.
Here, a lot of the most interesting solar physics takes place. It’s where the solar wind starts. It’s where loopy magnetic structures are anchored. It’s where space weather gets its start. And it’s where powerful ejections of mass become launched into space — ones that can knock out electric-power grids on Earth.
Today’s space telescopes can watch the outer corona all the time. They employ a disk called an occulter to block out the sun’s brightness. But it’s no match for a true solar eclipse. By lucky coincidence, the moon can appear exactly the right size in the sky to block out the solar disk, and no more.
Jay Pasachoff is an astronomer at Williams College in Williamstown, Mass. “The moon makes a better eclipse than humans have been able to do,” he notes. So he plans to take advantage of it. He will observe this year’s event — his 34th total eclipse — from Salem, Ore.
Story continues below image.
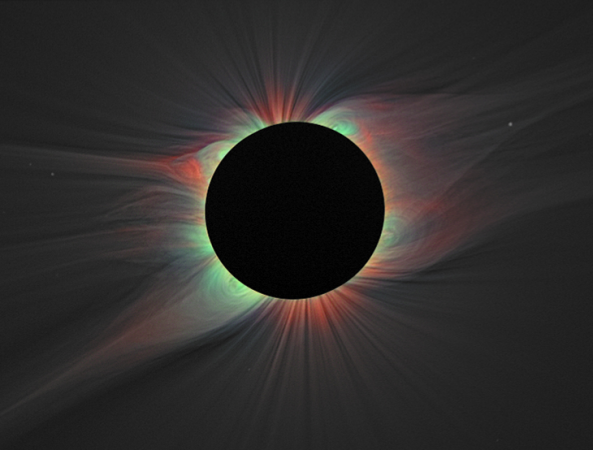
Where does the solar wind come from?
The sun can’t keep its hands to itself. A constant flow of charged particles streams away from it at hundreds of kilometers per second. That solar wind batters vulnerable planets in its path. Indeed, it is thought to have stripped away much of the Martian atmosphere. And it’s had a direct role in shaping life in the solar system. Our planet is protected from a fate similar to Mars’ only by its strong magnetic field. That field guides the solar wind around Earth.
But scientists don’t understand some key details of how this wind works. It originates in an area where the sun’s gaseous surface meets its atmosphere. Like winds on Earth, the solar wind is gusty. It speeds and slows in different areas. Right now, no one knows why the wind is fickle. But some answers might emerge during the eclipse.
Nat Gopalswamy is an astronomer at NASA’s Goddard Spaceflight Center in Greenbelt, Md. (NASA is short for the National Aeronautics and Space Administration.) He and his colleagues will test a new instrument, called a polarimeter (PO-luh-RIM-eh-tur). It was built to measure the temperature and speed of electrons leaving the sun. Measurements will start close to the sun’s surface and extend out to around 5.6 million kilometers (3.5 million miles). That’s about eight times the radius of the sun.
“We should be able to detect the baby solar wind,” Gopalswamy says.
The polarimeter will be set up at a high school in Madras, Ore. It will separate out light that has been polarized — had its electric field organized in one direction — from light whose electric field goes in all sorts of directions. Electrons scatter polarized light more than non-polarized light. So that observation will give the scientists a bead on what the electrons are doing. And that should offer clues to how fast the wind flows, how hot it is and even where it comes from.
The team first tried out an earlier version of this instrument in 1999. It was during an eclipse in Turkey. But that instrument required the researchers to flip through three different polarization filters to capture all the data on polarized light that they sought. Cycling through the filters using a hand-turned wheel was slow and clunky. It’s also a challenge when the totality lasts only two minutes or so.
The team’s upgraded instrument has been designed to gather data through all three filters and in four wavelengths of light at once. Because the corona morphs quickly, “we have to take these images as close in time as possible,” Gopalswamy explains. One exposure will take 2 to 4 seconds, plus a 6-second wait between filters. That will give the team about 36 images.
This isn’t the polarimeter’s first time in the field. Gopalswamy and his team took it all the way to Indonesia for a solar eclipse in March 2016. “That experiment failed because of noncooperation from nature,” Gopalswamy says. “Ten minutes before the eclipse, the rain started pouring down.”
This year, they chose Madras because historically it’s the least cloud-covered place on the eclipse path. But they’re still crossing their fingers for clear skies.
Why is the corona so hot?
The big star of the August 21 light show will be the sun’s corona. From its delicate and filmy look, you might expect it to be where the sun goes to cool off. That couldn’t be more wrong.
The corona is a mysteriously sizzling inferno. The sun simmers at about 5,500° Celsius (9,900° Fahrenheit) at its visible surface, the photosphere (FO-toh-sfeer). But the gas just above the photosphere runs some 10,000 °C (18,000 °F). Then, in the corona, the temperature makes an abrupt hike to several million degrees!
Paul Bryans is a solar scientist at the National Center for Atmospheric Research in Boulder, Colo. “It’s counterintuitive” — the opposite of what you’d expect — “that as you move away from a heat source, it gets warmer,” he notes. Why the corona should do that is “one of the longest unanswered questions in all of solar physics,” he says.
The corona is also diffuse. And that makes its scorching temps even stranger. The most basic ways to heat a material rely on particles crashing into each other. But the corona is too thin for that to work.
Such extreme temperatures must have something to do with the corona’s magnetic field. That is probably where all that energy is stored. Once there, the energy has a hard time escaping — radiating into space. So it just builds up.
“We know there’s energy coming in, and it’s hard to get it out unless you get very hot,” says Caspi. “What we don’t understand is how that energy gets into the corona in the first place.”
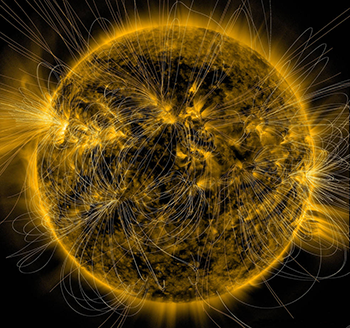
Physicists have several ideas. Magnetic field lines surround a magnet (you can see this if you drop iron filings around the edges of a bar magnet). Those field lines also exist in the corona. Maybe loops of them vibrate like guitar strings. That might heat things up, a bit like how a microwave oven heats food. Maybe the loops’ magnetic anchors on the sun’s surface braid and twist the magnetic field above them. That might dump in energy that is then continually radiated away (as from the heating element in a toaster).
Or maybe nanoflares or jets of solar gases — called spicules — carry energy away from the photosphere, dumping it into the corona. The formation of new coronal loops that connect to existing ones could dump in enough extra energy to heat up the plasma.
In search of answers, dozens of groups of scientists across the country will deploy telescopes equipped with filters during this year’s eclipse. They will attempt to pick out polarized light, infrared light or electron-deprived iron atoms. Bryans and his colleagues will be on a mountaintop near Casper, Wyo., in the path of totality.
Scientists have thought up many mechanisms that could contribute to the corona’s extreme heat. It’s difficult to declare just one as the single most important one. Ultimately, the solar eclipse is the best chance scientists will have to test them. It’s the only time the corona will be the star of the solar show.
What can the eclipse tell us about the corona’s magnetic field?
Corona is Latin for “crown.” And during an eclipse, the dazzling corona certainly gives every appearance that it’s crowning the sun. Its hot, bright plasma is a radiant, ever-changing spectacle. Its bright loops and whorls bend, sway and snap. Sometimes, one of those loops breaks off. That sends high-energy material rippling through space in what is known as a coronal mass ejection. If flung toward Earth, these bursts can trigger auroras. The energy deposited when they enter our planet’s atmosphere also can damage satellites and knock out electric-power grids.
It’s the motion of charged particles — like those in the corona’s plasma — that create the sun’s magnetic field. And it, in turn, choreographs the corona’s disordered dance.
In terms of the corona, the magnetic field, well, “it’s the heart of everything,” says Jenna Samra. She is a graduate student in applied physics at Harvard University in Cambridge, Mass.
That magnetic field at the sun’s surface is well known. But the corona’s field is so weak, scientists barely know it at all. Understanding the sun’s magnetic inner life could be key to understanding and predicting the corona’s dramatic activity.
During the 2017 eclipse, Samra and others will observe the corona at wavelengths (or radiation) between 1 and 4 micrometers — in the infrared portion of the light spectrum. These are light wavelengths emitted when a heavy element like magnesium, iron, sulfur or silicon loses an electron to the hot plasma around it.
Magnetic fields of different strengths make those electrons spiral in particular ways. That spiraling changes the orientation of the light as it travels toward Earth. In the end, to probe the magnetic field, scientists will have to measure this light orientation, or polarization.
Bryans and Philip Judge, who also works at the National Center for Atmospheric Research, will be part of a research team that trucks a sensitive instrument to the top of Casper Mountain in Wyoming. This device, a spectrometer (Spek-TRAH-meh-tur), can split sunlight into its component wavelengths.
“The [sun’s] whole infrared spectrum has never been acquired before. And that’s one of the things we want to do,” Judge says.
Samra will have another infrared spectrometer at an altitude of about 15 kilometers (9.3 miles). Her team, including her adviser Edward DeLuca, and their instrument will take off on a jet owned and flown by the National Science Foundation. Water in Earth’s atmosphere absorbs some of the infrared wavelengths they’re interested in. The extra height will get them above most of that water. They’ll fly east from Chattanooga, Tenn., in the shadow of the moon. That will extend the eclipse a bit for them. The team will get four minutes of the moon entirely blocking the sun. This should give them more time to collect data.
The spectrum these teams measure during the eclipse won’t translate immediately to a measurement of the strength or shape of the sun’s magnetic field. But it will help identify which wavelengths are easiest to observe. That in turn will set the stage for the work of future telescopes, like the Daniel K. Inouye Solar Telescope. It is under construction on the Hawaiian island of Maui, and due to start up in 2019.
“Half of its mission will be to observe the infrared and the [solar] corona. But right now we don’t know what it should look for,” Judge says. Measurements made during this year’s eclipse will help point the way.
Story continues below image.
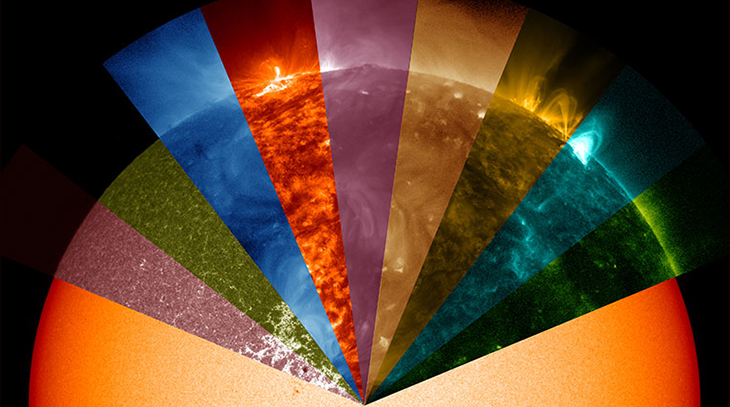
Why are the loop structures in the corona so organized?
During the eclipse, the corona’s orderly network of loops, fans and streamers become visible. These wispy structures arise from the magnetic field on the sun’s visible surface.
“It’s not a static surface like the ground,” explains Amir Caspi. “It’s more like an ocean,” notes this solar physicist at the Southwest Research Institute in Boulder, Colo. “And not just an ocean. It’s like a boiling ocean!”
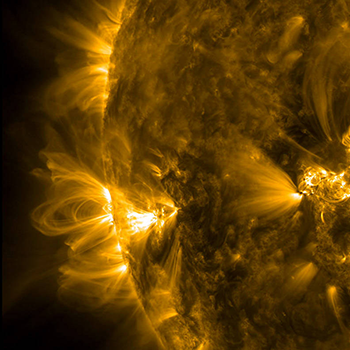
Because the corona’s loops and streamers all originate at the turbulent surface, their roots should get twisted and turned around. “And yet these structures in the corona are not tangled and snarled and matted like kelp or seaweed in the ocean,” Caspi says.
And, he adds, “Nobody understands why.”
To unknot the photosphere’s tangled mats, the corona must somehow release some of the energy stored there, Caspi says. So during the eclipse, he and colleagues will be looking for the release valves that set the corona free.
One possibility is that the wave motion in the corona’s magnetic field lines helps untie the snarls. Magnetic waves in plasma, called Alfvén waves, are thought to ripple through the sun’s magnetic field lines like vibrations in a guitar string.
Another option is that little puffs of magnetic energy near the solar surface could help release the tangles. These puffs are called nanoflares and nanojets. No one knows if they exit. But scientists suspect that they might. They would be like solar flares but with only a billionth as much energy.
By going off all the time, nanoflares and nanojets might collectively release enough energy to give the corona some structure, computer models indicate.
“Both are symptoms of tiny rearrangements of the magnetic field,” says Craig DeForest. He’s a solar physicist, also at the Southwest Research Institute. Solar flares and coronal mass ejections are signs of such magnetic rearrangements. But they don’t occur often enough to account for the corona’s smoothness. “Nanojets and/or nanoflares in the middle corona would be a smoking gun,” DeForest says. They could “explain why the corona is so organized.”
Researchers have directly observed Alfvén waves in the lower corona, within about half a solar radius of the surface. But they’ve not been seen farther out where waves with different energies would travel. And no one has actually seen any nanoflares or nanojets. Theories suggest they may just be too small and quick to see individually. But they should be visible as an ensemble when the solar eclipse reveals the lower corona.
Caspi, DeForest and their colleagues will fly a pair of telescopes on a NASA WB-57 high-altitude research jet in the path of the eclipse. They hope to see both effects during the eclipse. “We’re taking high-speed movies of the sun and analyzing them for things that look like waves,” Caspi says. “We’re just overall looking at the structure of the corona.”
So it may seem as if an eclipse is little more than Mother Nature’s ultimate light show. In fact, it also can be a great environment to probe some great remaining questions about the workings of our home star.